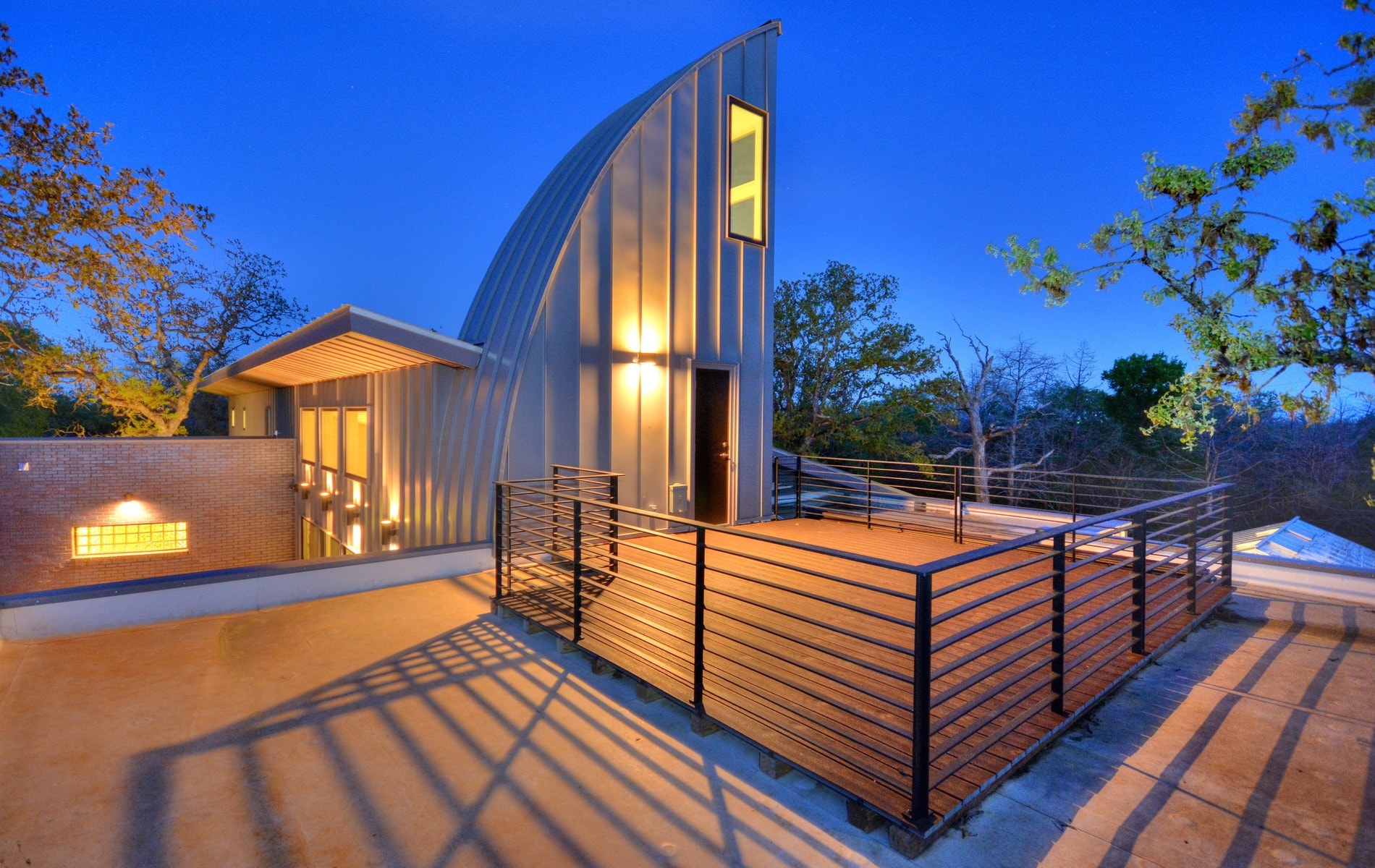
vie-magazine-future-of-cad-hero-min
The completed project with the thermal chimney in the foreground, as seen from the rooftop deck. Photo by Cris Diaz, Shutterbug Studios.
Transforming How Buildings
are Designed with Software
By François Lévy
Not surprisingly, we architects typically have a deep regard for the physical form of a building, accompanied by a fundamental urge to create satisfying forms. Of course, what constitutes “satisfying” may be a function of personal taste, cultural expectations, professional norms, or even what is merely fashionable. Given too much free reign, a concern for pure form threatens to overwhelm the overall design and may lead to self-indulgent solutions that prioritize form over solving for design constraints such as the building program, homeowner expectations, the application of appropriate building technologies, cost, building performance, durability, and so on. In other words, a disproportionate concern for the idiosyncratic appearance of a building risks overwhelming the function and utility of the project. And paradoxically, the outward architectural expression will suffer along the way.
There is design as a noun, and then there is design as a verb. When architects start with a pre-conceived form, and try to shoehorn solutions to design problems, the end result is rarely as pleasing as when the architectural form arises from the constraints of the project itself. To design is to solve a problem, and not just for one variable; at it’s most challenging to design is to reconcile the irreconcilable. A simple design problem has by definition one or more obvious solutions. When design is a process worthy of thoughtful consideration, it addresses complex or unseen problems. When a design problem has only one constraint, or when all constraints point in the same direction, the design solution is self-evident. When multiple constraints compete with one another, contradict one another, clash with one another, then there arises an opportunity for a new richness to result, an architectural expression that solves pragmatic problems and is surprising, expressive and delightful.
I would argue that there are three fundamentals to the design process. First, it’s incumbent upon the designer to thoroughly understand the particular individual constraints of a given design problem. Second, the designer must uncover those areas where constraints conflict. Third, in the resolution of those constraints there emerges an opportunity for the unexpected. Like most creatives, I know that I’m on to something when an unforeseen solution surfaces, one of which I had no inkling when I began to work. There are many instances when a design solution seems to come from somewhere else. While it might have subconsciously been with me all along, I suspect that instead, surprising solutions arise out of an immersion in understanding the project’s constraints. In architectural practice today, we have digital design tools that if used properly can help designers better understand project constraints and quantify their conflicts, leading to richer, more satisfying outcomes.
Building Information Modeling and Boussoleil
For a couple of decades now, architecture has increasingly been designed on building information modeling software platforms, or BIM. BIM involves the creation of data-rich 3D models, and all the construction documents (working drawings) are derived from that model. In the older tradition of computer-aided design/drafting (CAD), and hand drafting before it, the “model” was in the architect’s mind, and the drawings were two-dimensional “views” of that model. A builder, consultant or homeowner would have to interpret each 2D drawing individually, then mentally reassemble the set of drawings into a “model” for themselves. Naturally, that it’s challenging for someone without training to correctly interpret drawings, and it’s no wonder that many non-architects are a bit surprised when they see the final space, having had to interpret a set of somewhat cryptic drawings. With BIM, the model is created first, and 2D views (or drawings) are extracted from it. Not only that, but the objects in the model are intelligent: doors “know” that they are doors, and can be inserted into walls, and those walls have wall-like properties, like insulation value, or fire resistance, sound transmission, and even cost. Models are much easier for homeowners and builders to visualize (and architects, too), and while setting up the model ahead of time can be a bit more time-consuming, generating drawings from it is much more efficient. In addition, changes to the model—and there are an indefinite number of changes possible on the design process—are directly reflected in the drawings, which helps avoid errors. Architects thus spend less time managing their drawings, and more time focused on designing for their clients. Homeowners benefit directly, with models, animations, and renderings that are far more eloquent than traditional working drawings.
As mentioned above, BIM objects are data-rich, and as a result there are opportunities for architects to capitalize on that information to quantitatively validate our traditionally qualitative design, enriching the architectural outcomes and creating buildings that are more responsive to homeowner needs, climate, and performance. Boussoleil, (a portmanteau from the French words for “compass” and “sun”) is a recently completed 2,500 square foot single-family residence that our firm designed for a site in rural Central Texas (figure 1). This case study illustrates the design possibilities of data use in digital building models.
Boussoleil. The project is located on a 16-acre site with moderate topography and a significant population of mature, indigenous oaks. The homeowner wanted the home to be as energy efficient as possible, with minimal maintenance and operational costs, and with a concern for aging in place. Minimizing eastern and especially western exposure to control solar heat gain was an appropriate response to local climate. By modeling the earliest design iterations using Vectorworks Architect software, a BIM authoring tool, we optimized the building orientation and the roof design to minimize solar heat gain in warm months, while maximizing the potential for onsite solar photovoltaic (PV) energy production. We used a virtual heliodon tool to conduct a variety of sun studies, both static and animated (figure 2), so we could design glazed openings to keep the home cooler in summer and warmer in winter (contrary to popular perception, Texas winters can at times be chilly, especially in the country). Simultaneously, we located the solar array to avoid shading from neighboring trees and roof features—see also figure 5 below.
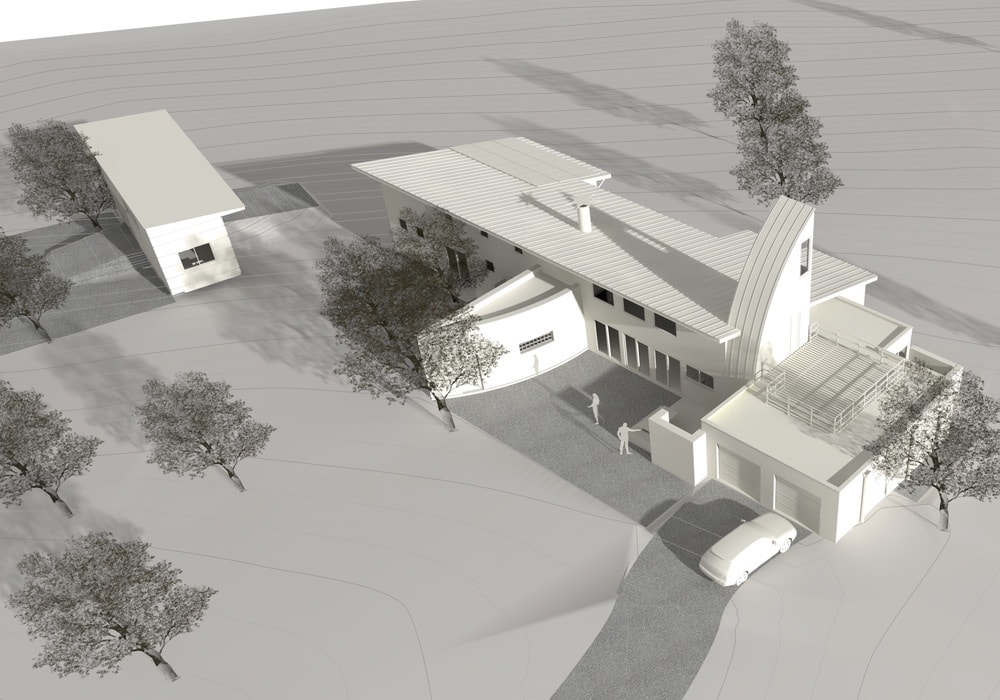
A white “museum board” rendering of the Boussoleil project, emphasizing the solar-optimized roof and curved thermal chimney. A small detached studio and guest cottage is oriented facing true south, top left. This rendering (as well as the others in this case study) were produced in Vectorworks Architect software, a BIM-authoring application.
Another project requirement was that the floor be on a single level with no steps, in order to address the owners’ goals for aging in place. The ground at the selected building site sloped significantly, such that a true orientation to the cardinal points would not only increase costs but would also have a significant portion of the building well over six feet out of grade. By once again employing the quantitative information available in BIM, a variety of slight variations in orientation of the building and their effects on solar shading could be studied and compared to corresponding ground cut-and-fill calculations derived from the BIM site model. In the end, an orientation that was 15° east of south was a good balance between minimal loss of solar collection while reducing site work costs and maintaining the owner’s goals.
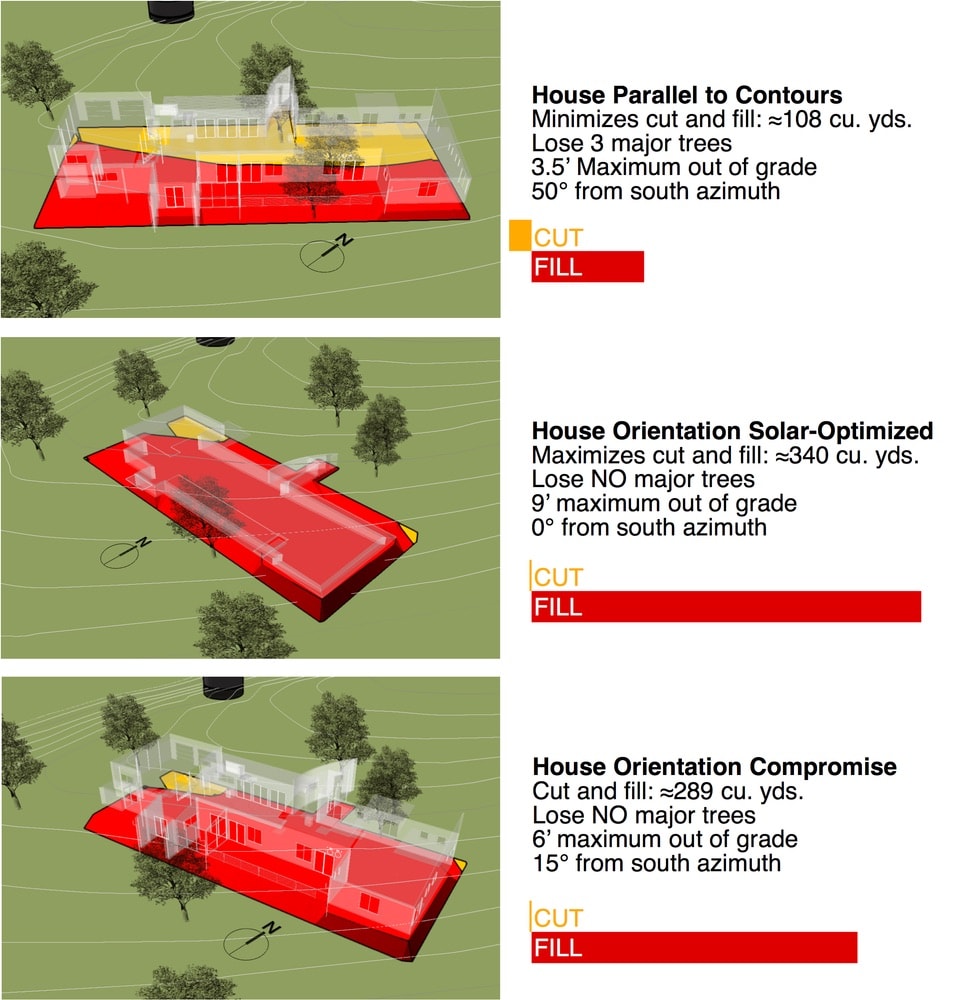
Even on a 16-acre site there isn’t complete freedom to site a building for optimum photovoltaic collection. These diagrams illustrate the quantitative analysis undertaken to negotiate competing site work concerns—minimizing cut and fill, preserving trees, and maintaining an accessible floor plan—without unduly compromising solar shading and PV collection. Here, the analytical opportunities of a BIM site model and solar modeling helped satisfy both concerns.
Too Much Too Late? Energy modeling is a critical design factor for many projects, but proper energy modeling requires detailed technical data that may not be known early in the design process. By the time that information is available, it may be too late to make significant improvements to the project’s performance. Yet as with most aspects of building design, energy performance should be considered in early design. Fortunately, architects have BIM energy analysis (as distinct from energy modeling) tools at their disposal. Unlike energy modeling, which requires the detailed information mentioned above, energy analysis makes more general, yet valid assumptions and calculations concerning building performance.
Commonly available built-in BIM tools like Vectorworks’ Energos allow the designer to make conceptual energy analyses at early design phases. For example, the architect can stipulate wall insulation amounts as prescriptive targets in early design, then substitute specific wall assemblies for generic ones as the design is developed. Simply by substituting one wall type for another, competing hypothetical scenarios can be compared and the benefit of a proposed change evaluated. This ensures code compliance or a high-performing energy efficient home from the start. By the time granular energy modeling is deployed, the design has already been vetted at a coarser level, avoiding the pitfall of “too much analysis too late.” Architects who employ these methods from early design can avoid designing homes for their clients that are expensive to heat and cool, or require extraordinary last-minute design changes or expensive windows, doors, and wall systems to compensate for poor energy performance.
Resolving conflicting constraints: thermal chimney and PV. A fundamental principle of energy-efficient design is to reduce energy loads first, then seek ways to expend energy more efficiently when necessary, and finally to find ways to appropriately generate alternatives to fossil fuels. In hot, humid climates like that of Central Texas, ventilation is the most effective passive cooling strategy, as it displaces the human psychrometric comfort zone into a warmer, more humid area of the chart. For those days when there is no wind-driven airflow, Boussoleil employs a thermal chimney to naturally ventilate the house, exploiting the stack effect, warm air’s natural buoyancy. This passive cooling technique is centuries old: warm air rises, is evacuated from a high aperture, and entrains lower, cooler air to move throughout the space. But how tall should the thermal chimney be? How much glass should it have? There are well-established design guidelines such as those developed by the American Society of Heating, Refrigerating and Air-Conditioning Engineers (ASHRAE) that allow the designer to estimate passive stack effect air flow rates based on the design of the building. The ASHRAE approximation is a fairly simple algebraic equation that we incorporated in a spreadsheet embedded within the project BIM file. As a result, we could explore alternative thermal chimney designs and instantly evaluate them for their performance, not just appearance (figure 3).
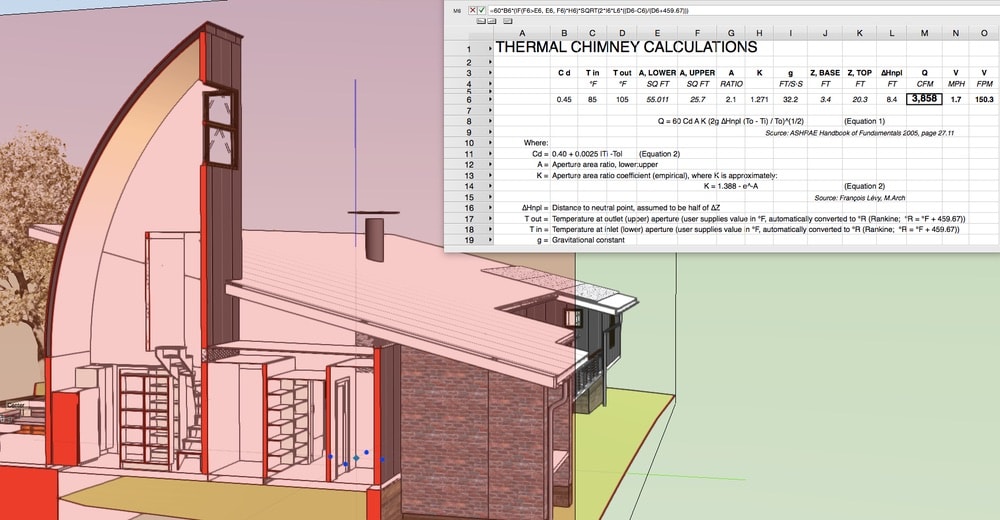
The thermal chimney’s east, south, and west orientations are heavily glazed to encourage heating its upper air, which entrains lower, cooler air as it rises and is exhausted from the south window. The author’s thermal chimney calculation worksheet is integrated in the BIM project file to help assess the performance implications of variations of design variables, primarily thermal chimney height and inlet and outlet aperture areas.
The taller the thermal chimney, the greater the air flow it can produce, but the longer the shadow it casts—literally—on the photovoltaic array. While PV controls have improved in recent years to help mitigate shading losses, partial shading of an array is still undesirable and disproportionately affects its overall performance. To resolve this conflict, we designed a quantitative analysis process using the information from the virtual building. Using the data from the BIM model combined with solar animations, we refined the design of the thermal chimney to have a smaller profile while maximizing airflow rate and avoiding shading the PV array. Simultaneously, we used digital tools to find the ideal PV array size and placement based on climate data and the available shade-free roof.
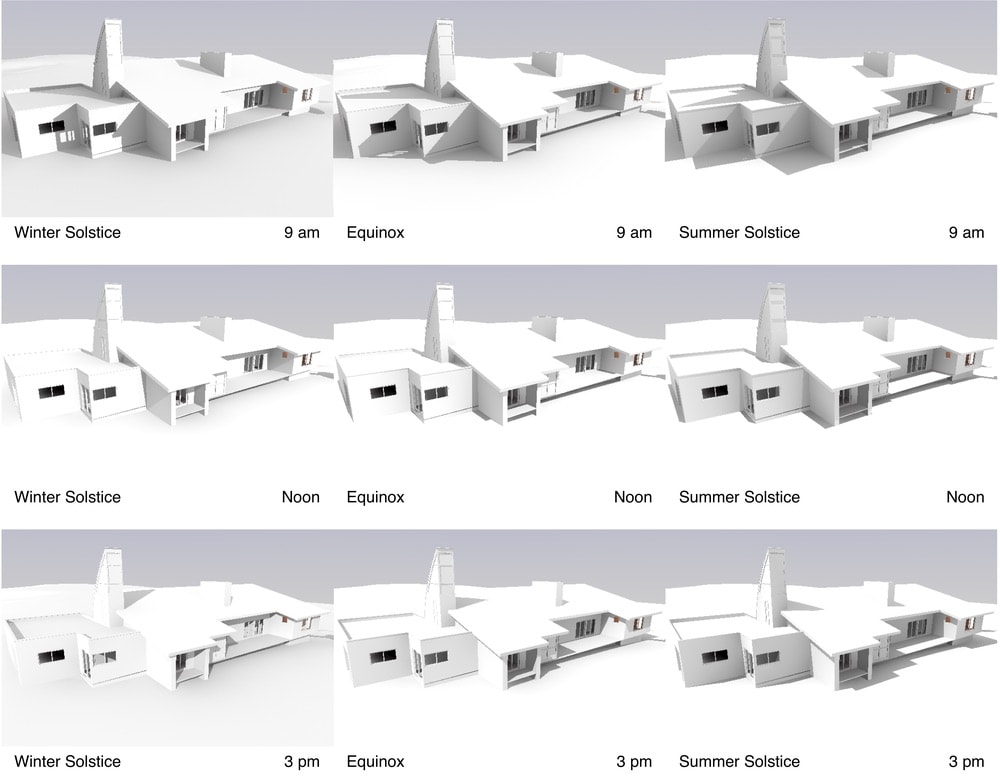
These sun studies are representative of the types of solar animations undertaken to ensure that various solar chimney configurations would not interfere with photovoltaic collection throughout the year.
Rainwater harvesting. Regionally, rural residences have required deeper and deeper water wells over the last two decades, in part because of changing weather patterns but also due to the encroachment of suburban development, which stresses and lowers the water table. For such projects, harvested rainwater has become an increasingly attractive water source for irrigation as well as for domestic use. While BIM authoring software does not include a “rainwater harvesting tool,” it is possible in most of these applications to link available projected roof area to rainfall data in order to size water storage tanks, or cisterns, the single most expensive component of a rainwater system (figure 6). The advantage of including these calculations in the BIM file is that tank size can be determined at any step of the design process. This allows the designer to rapidly weigh the architectural impact on the project of various roof designs and their dependent cisterns.
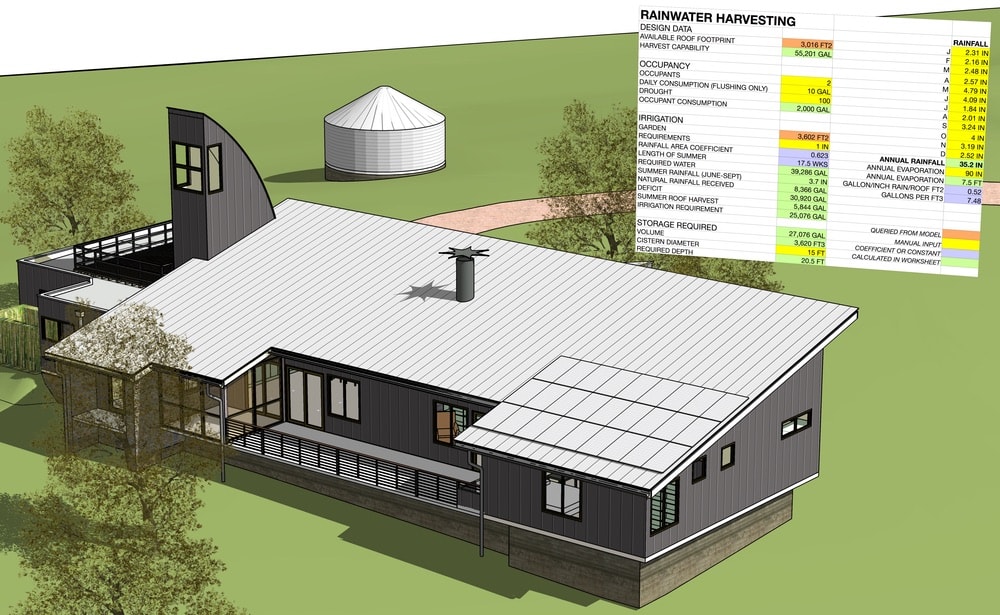
The roof in the Boussoleil BIM project file is an “intelligent” object, able to report its actual surface area (amount of roofing material) as well as its projected area (footprint in plan view), and distinguishing area over conditioned space from overhangs. Here, a custom worksheet queries the plan projected roof area in order to calculate the required cistern size. Accurate modeling of the building’s site, tree canopies, and the elements of the design itself help maximize the PV array’s unobstructed solar access.
Conclusion
Architects often refer to “design problems,” and that is an apt term. Design problems are often quite complex, and are required to resolve competing agendas of site, climate, context, program, use, aesthetic and architectural concerns, constructability, permitting, durability and cost.
Good design solves problems. Great design solves them elegantly and beautifully. Like any problem solver, an architect is most successful when good data is available. BIM helps provide that data; not just about the conditions of the design (site restrictions, solar geometry, and so on), but also feedback about the proposed design solution itself. When the architect capitalizes on that opportunity, queries the evolving the model, and weighs the resulting information against other, architectural judgments, then the resulting design is richer and likelier to solve more aspects of the design problem. The architect might rely on intuition informed by experience, but such intuition may at times be faulty, even when well informed.
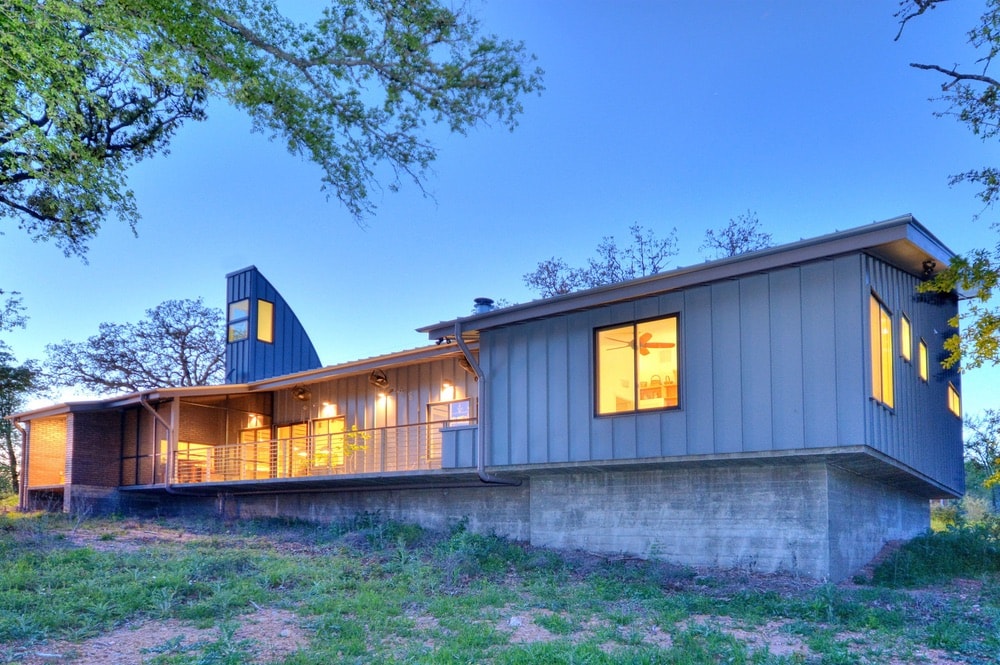
Boussoleil. Photo by Cris Diaz, Shutterbug Studios
When properly used, the data inherent to BIM allows for projects that are more closely indexed to site conditions, climate, and solar geometry. The quantitative assessment of materials used may likewise permit the architect to better evaluate the effect on resources of competing design solutions. Such a computational design process can lead to better-performing architectural projects. This is especially the case for smaller buildings, for which external energy loads (that is, climate loads) have a more significant impact on performance than internal ones. For homeowners, intelligent digital design processes that take full advantage of BIM can lead to buildings that perform better, are responsive to and respect their environment, cost less to operate—and may be more beautiful.
— V —
Share This Story!
KEEP UP WITH THE LATEST STORIES FROM VIE